Professor
Ph.D., Massachusetts Institute of Technology (1999)
Current Research
- Epitaxial Oxides on Semiconductors
- Scanning Tunneling Microscopy Investigations of Organic/Semiconductor Interfaces
- Tunneling Spectroscopy for Molecular Electronics and Nano-Sensors
- Nanoscale Investigations of Electrocatalysis
Professional Activities
Coordinator: AIChE Area 8E, Salt Lake City, UT, 2010
Session Chair: AIChE, Nashville, TN, 2009
Member: Materials Research Society
Member: American Institute of Chemical Engineers
Session Chair: AIChE, National Meeting, Philadelphia, PA, 2008.
Session Chair: AIChE, National Meeting, San Francisco, CA, 2006.
Session Chair: AIChE, National Meeting, Austin, TX, 2004.
Current Research Group
Ph.D. Students | M.S. Student | Undergraduate Students |
Han Wang Xiaoqiang Jiang Kan Fu |
Nan Li | Chris Hawkhurst David Robitaille |
Research Statement
Epitaxial Oxides on Semiconductors
There is tremendous interest in the growth of nanometer thin oxide layers on semiconductor substrates for applications in microelectronics and nanotechnology. In particular, epitaxial oxides, i.e. oxides grown as single crystals on semiconductor wafers, are attractive for nanoelectronic devices that harness the functional properties of the perovskite oxides such as BaxSr1-xTiO3 (BST) and PbxZr1-xTiO3 (PZT).
Promising applications include nanomachine memory devices that write data bits with arrays of AFM cantilevers using the ferroelectric properties of PZT. The technological realization of these device concepts requires that high quality epitaxial oxide layers can be grown in a cost effective manufacturing process. Over the past 10 years, there has been a rapid advance in the ability to grown epitaxial oxides on silicon wafers using growth methods based on molecular beam epitaxy (MBE). MBE is a growth technique based on tiny fluxes of atoms slowly arriving and incorporating onto a heated surface in ultra-high vacuum. MBE can be used to grow very precise layers, but scaling MBE growth reactors for manufacturing is difficult due to the slow growth rates, high capital equipment cost, and complex reactor operation. It would be a significant advance for the application of epitaxial oxides if a chemical deposition process such as chemical vapor deposition (CVD) or atomic layer deposition (ALD) could be invented.
We are investigating the growth of epitaxial oxide layers on semiconductors by CVD and ALD methods. CVD and ALD replace the atomic fluxes used in MBE with chemical reactants that are transported to the growth surface in the vapor phase and decompose via chemical reactions to grow metal-oxide layers on the semiconductor surface. The CVD/ALD processes are more favorable for manufacturing, but they add considerable complexity due to the chemistry of the deposition process. In addition, the growth of epitaxial oxides requires strategies to nucleate the oxide layers on the semiconductor substrate without oxidizing the substrate. Achieving these goals requires an unprecedented level of understanding of the chemical processes at the growth surface. We use in-situ tools including spectroscopy, diffraction, and microscopy to investigate the growth of nanometer oxide layers one layer at a time. The attached figure demonstrates a low energy electron diffraction (LEED) image of a (3×2) Sr/Si(100) layer grown using ALD from β-diketonate precursors and ozone as the oxidan
Scanning Tunneling Microscopy Investigations of Organic/Semiconductor Interfaces
A common theme among emerging nanotechnologies is the desire to integrate biological and/ or molecular structures with inorganic materials to make hybrid devices. In particular, the alignment of the metal or semiconductor Fermi level with organic molecules and the mechanism of charge transport across the interface is an active area of investigation. Interface charge transfer is important to new concepts for energy conversion and molecular devices. Semiconductors offer several advantages over metals due to covalent bonding and the ability to adjust the Fermi level with doping. Fundamental to these investigations is the requirement to understand, predict, and ultimately control the chemical bonding of molecules at semiconductor interfaces. The “mechanistic rules” for adsorption are required to design surfaces for useful properties, and even small areas of defects or variant order may disrupt the desired functionality.
Scanning Tunneling Microscopy (STM) and related Scanning Tunneling Spectroscopy (STS) are powerful experimental tools to investigate molecular adsorption and molecule-surface charge transport at the single molecule scale. The attached figure demonstrates an example of molecular adsorption on the Si(100)-2×1 surface. The bright protrusions are found to be tertiary butyl regions of the adsorbed molecule, 2,2,6,6-tetramethyl-3,5-heptandionate (tmhd), projecting away from the surface. Analysis of the images with computational chemistry methods demonstrates that the molecule preferentially reacts through the hydroxyl group (-OH) present on the molecule. Minority products from other adsorption pathways are also present, showing a lack of selectivity for the adsorption mechanism. Present work is concentrated on Si, but future work will expand to other semiconductor surfaces including Ge, III-Vs, and wide band gap materials. Objectives are to design chemical processes (including molecules and surfaces) for ordered adsorption with desired electrical, mechanical, or optical properties.
Tunneling Spectroscopy for Molecular Electronics and Nano-Sensors
Molecular electronics is an exciting new field of research that seeks to understand charge transport and chemical bonding at the single molecule level. Such knowledge may create active nanostructures that extend computing, electronic memory, bio-chemical sensing, or energy harvesting beyond the limits of modern technologies. The research holds great promise due to the richness of molecular properties and the complexity of inorganic, organic, and biological molecules that can be synthesized. One of the most intriguing applications of molecular electronics is to fabricate nanosensors based on molecular recognition for sensing applications ranging from homeland security to medical diagnosis or forensics.
Our work is a novel experimental approach that uses tunneling spectroscopy as a methodology to investigate the chemistry of single molecules in nanoscale junctions. We have invented a device that can trap molecules between nanoelectrodes in nanoscopic tunnel junctions and probe the chemical bonding with inelastic electronic tunneling spectroscopy (IETS). The attached figure shows IETS spectra of normal and dueterated acetic acid molecules adsorbed in a copper nanojunction. The IETS spectra shows peaks at molecular vibrations (similar to infrared spectroscopy), which helps us understand how the molecules are configured in the tunnel junction. We can also observe reactions such as adsorption, desorption, and bond breaking. These molecular events are detected as a change in the tunneling signal, and reactants and products are characterized using vibrational spectroscopy at the single molecule scale.
Our goals are to use nanofabrication principles to control the electrode and tunnel junction properties for arrays of billions of parallel tunnel junctions that can serve as an “electronic nose” to sense molecules from the environment. Achieving these goals requires subnanometer precision for engineering the electrodes with 0.1 nm accuracy. We use atomic layer deposition (ALD) and advanced reaction engineering principles to control the nanoelectrode microstructure and electrical properties to achieve electrodes spaced less than 2 nm apart. Scaling up the nanostructures to arrays of billions of parallel devices requires understanding gas phase transport, surface transport, and surface reactions at the nanometer scale. These processes are complicated by the application of strong electric fields to help trap and orient molecules in the junction. We are interested in understanding how molecules bond to the electrodes and each other in these confined spaces. Such knowledge will enable us to engineer selective interactions between molecules for mimicking the biological sense of smell. In addition, using different electrode materials allows us to investigate surface reactions of interest to a broad spectrum of surface science applications in areas such as catalysis and solar energy research.
Nanoscale Investigations of Electrocatalysis
Electrocatalyst durability is one of the main hurdles facing the development of fuel cell technology. A loss of catalytic activity from the degradation of the Pt/support interface is one of the main failure mechanisms of PEM fuel cells. Our research program is investigating the mechanisms of degradation at the nanoscale through a combination of scanning tunneling microscopy and electron microscopy with electrochemical measurements of model electrocatalysts. Degradation of the electrocatalytic activity is observed at the nanoscale where Pt particles detach from the surface and aggregate into larger particles. The attached figure illustrates the change from dendritic aggregates of 2-3 nm Pt particles that cover the carbon surface to larger particles and lesser surface coverage after 10 hours of cycling 0 – 1.0 V (NHE). This work seeks to develop modifications of the support layer to stabilize the Pt nanoparticles against loss of electrocatalytic activity. The methodology is to investigate the Pt-Pt and Pt-support interactions at the nanoscale and develop theoretical models that capture the essential physical and chemical processes that lead to degradation. Through this research program, we are investigating alternative catalyst and support materials to improve the durability of electrocatalyst formulations.
Previous Positions
2002-2008 | Assistant Professor of Chemical Engineering, University of Delaware. |
2001-2002 | Agere Systems, High Speed Devices and Interfaces Laboratory, New Jersey. |
1999-2001 | Bell Laboratories (Lucent Technologies), Silicon Device Research Laboratory, New Jersey. |
1993-1999 |
Graduate Research Assistant, Massachusetts Institute of Technology
|
Awards & Honors
2007 | Nanotech Measurement Contest (sponsored by Keithley Instruments, Inc.), 2nd place (with graduate student R. Gupta) |
2005 | Emmert Faculty Fellow 2003: National Science Foundation Career Award |
1992 | Dow Award for 1st Course in Chemical Engineering |
Recent Publications
Han Wang, Xiaoqiang Jiang, Kan Fu, and Brian. G. Willis*, Nucleation, Hydroxylation, and Crystallization Effects in ALD SrO, J. Phys. Chem. C, 2013, v.117, pp. 11578−11583
Hsu, I. J.; Kimmel, Y. C.; Jiang, X. G.; Willis, B. G.; Chen, J. G., Atomic layer deposition synthesis of platinum-tungsten carbide core-shell catalysts for the hydrogen evolution reaction. Chemical Communications 2012, 48, (7), 1063-1065.
Wang, H.; Jiang, X. Q.; Willis, B. G., Real-time spectroscopic ellipsometric investigation of adsorption and desorption in atomic layer deposition: A case study for the strontium bis(tri-isopropylcyclopentadienyl)/water process. Journal of Vacuum Science & Technology A 2012, 30, (1).
Weiland, C. R.; Yang, L.; Doren, D. J.; Menning, C. A.; Skliar, D.; Willis, B. G.; Chen, J. G. G.; Opila, R. L., Binding of styrene on silicon (111)-7 x 7 surfaces as a model molecular electronics system. Journal of Vacuum Science & Technology A 2012, 30, (3).
Hsu, I. J.; Kimmel, Y. C.; Jiang, X.; Willis, B. G.; Chen, J. G., Atomic Layer Deposition Synthesis of Platinum-Tungsten Carbide Core-Shell Catalysts for the Hydrogen Evolution Reaction, Chem. Commun. 2012, 48, p. 1063-1065.
Hsu, I. J.; Hansgen, D. A.; McCandless, B. E.; Willis, B. G.; Chen, J. G., Atomic Layer Deposition of Pt on Tungsten Monocarbide (WC) for the Oxygen Reduction Reaction, J. Phys. Chem. C 2011, 115, 3709.
Wang, H.; Jiang, X.; Willis, B. G., Real-time Spectroscopic Ellipsometric Investigation of Adsorption and Desorption in Atomic Layer Deposition, J. Vac. Sci. Technol. A 30(1), (2011).
Willis, B. G.; Zhang, C. B.; Wielunski, L., Formation of Strontium Template on Si(1 0 0) by Atomic Layer Deposition, Appl Surf Sci 2011, 257, 4826.
D. B. Skliar and B. G. Willis, “The role of dangling bonds in H2O induced oxidation of Si(100)-2×1,” J. Phys. Chem. C, v. 112, p. 9434 (2008).
B. G. Willis and A. Mathew, “Growth of ordered SrO layers on Si(100) using metal-organic surface reactions,” J. Vac. Sci. Technol. A, v. 26, p. 83 (2008).
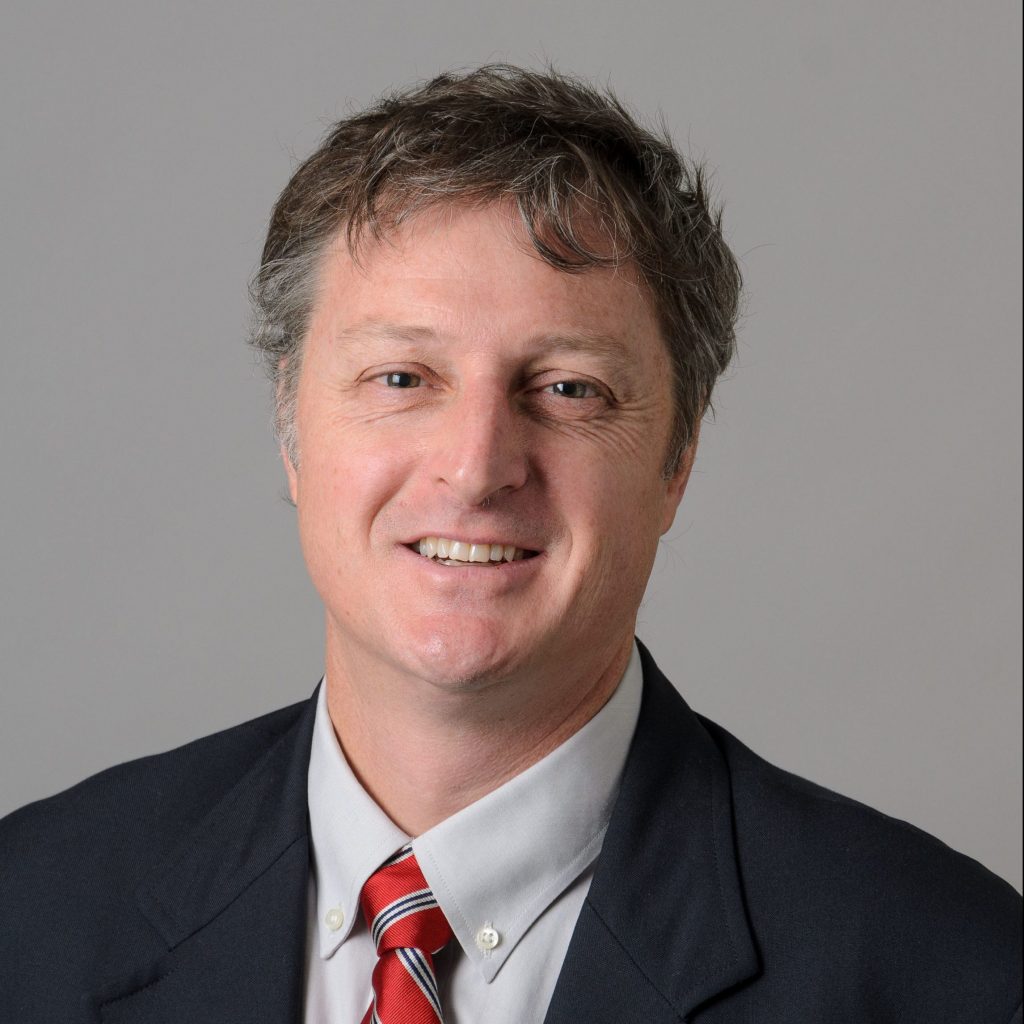
brian.willis@uconn.edu | |
Phone | (860) 486-9429 |
Mailing Address | 191 Auditorium Road, Unit 3222, Storrs, CT 06269-3222 |
Office Location | UTEB 288 |
Link | http://willislab.research.uconn.edu/ |